The Mitochondrion Pt. 2 — The Electron Transport Chain
We will be skipping over a detailed description of the Krebs (or TCA) cycle temporarily to talk about ATP production on the inner mitochondrial membrane. This is perhaps the most important function of the mitochondria–each molecule of ATP/ADP travels between the mitochondria and the cytosol approximately once a minute. Each day, 2 x 1016 molecules of ADP are phosphorylated in our bodies: 160kg/day. Each ATP Synthase complex can phosphorylate up to 100 molecules of ADP per second. This phosphorylation is a chemiosmotic process, or driven the flow of ions across a selectively permeable membrane. In this case, the ions flowing are protons. A concentration, charge and pH gradient is set up across the inner membrane, and the osmotic pressure to return to equilibrium is used to synthesize ATP from ADP.
However, in order for a gradient to be taken advantage of, it must first be established. The way the mitochondria sets this up is through the electron transport chain. The big picture here is that high energy electrons created by the TCA cycle are used to reduce molecules of O2 into water, and the energy given off is used to drive a series of proton pumps pumping protons out of the matrix space and into the intermembrane space of the mitochondria. These protons are then let back in by ATP synthase, driving the phosphorylation of ADP into ATP. It is important to note that the electron transport chain consists of the transfer of the high energy electrons through more than 60 different electron carriers. The change in energy from the point at which they are produced to the point where they reduce molecular oxygen is so great that the energy must be carefully lowered by successive transfers in order to keep the release of energy from being too explosive. The directionality of the chain is produced by each successive transporter molecule having a higher affinity for the electrons than its predecessor. Thus the chain allows 50% of the energy in the electrons to be captured in productive work, the other half being released as heat energy.
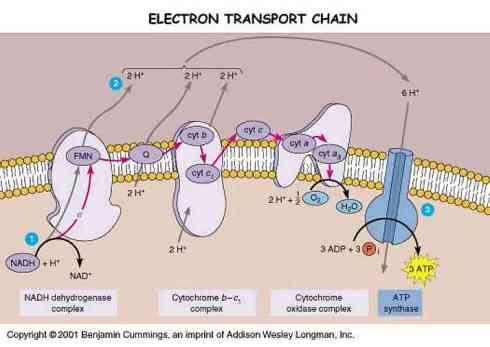
Why is oxygen the end for the electron transport chain? Well, molecular oxygen is very electronegative, and thus a lot of energy can be take from the electron before it is handed over to oxygen. However, the end point in the electron transport chain does not have to be Oxygen. Anerobic bacteria use almost the same process to catalyze production of ATP as eukaryotes, but end up depositing the electrons on other electronegative species such as as Sulfur and Nitrogen. It was only the rapid accumulation of atmospheric oxygen 1.6 billion years ago that made it possible for this process to end in the reduction of molecular oxygen.
Let us first focus on the electron transport chain. For each molecule of Acetyl-CoA the Krebs cycle produces 5 molecules of NADH, one molecule of FADH2 and one molecule of GTP. NADH and FADH2 are both electron carriers that are used as inputs to the electron transport chain and NADH is probably the most important. NADH is the reduce form of NAD+, which is free floating in the matrix space. NADH will diffuse to the edge of the membrane, where it will eventually associate with the first proton pump, NADH Dehydrogenase (also called Complex 1). NADH Dehydrogenase is a complex of more than 40 different polypeptides. Most of these polypeptides are themselves electron carriers, mostly of the iron-sulfur center variety.
Each NADH carries two electrons. These electrons are released one at a time to Complex 1, and each pulls two H+ ions through the complex to the other side of the membrane before it is passed off, for a total of 4 H+ from each NADH that is dehydrogenated by Complex 1. The electrons are then passed off to the second free electron carrier in the electron transport chain, Ubiquinone.
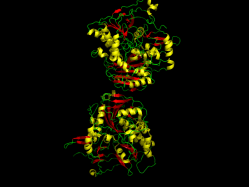
Of the 60+ electron carriers in the electron transport chain, only NADH, Ubiquinone and Cytochrome C are not part of one of the four large electron transport complexes. While Ubiquinone (also known as coenzyme Q) is not a part of one of the major complexes, it is implanted in the membrane. Ubiquinone is a freely diffusing molecule within the membrane, acting as a transporter. Part of the beauty of this system is that while each electron travels along a specific path from type of complex to next type of complex, if one complex becomes damaged, the electrons being ejected from a previous complex are not stalled–they simply are carried by Ubiquinone to another instance of the same complex.
Ubiquinone picks up the two electrons originally from NADH and transfers them to complex 3 (there is a complex two, but it is not part of the NADH electron transport chain), also referred to as the cytochrome b-c1 complex. Complex three is smaller than complex one, containing only 11 polypeptide chains, and has carriers predominantly of the cytochrome variety. On their way through complex 3, each electron pulls two H+ across the membrane, for a total of 8 protons thus far per molecule of NADH.
Cytochrome C is the next free floating electron transporter. Cytochrome C binds the electrons it carries via a heme group, and travels on the outside of the inner membrane to complex 4, the cytochrome oxidase complex. The cytochrome oxidase complex is slightly larger than complex 3, as it is a dimer containing 13 polypeptides. The transfer of each electron through this complex pulls 1 proton through the complex to the intermembrane space, for a total of 10 protons per original molecule of NADH from the Krebs cycle. This is the last stop on the electron transport chain. At this point, the electrons are transferred four at a time to molecular oxygen, to make two molecules of water.
However, NADH is only one of the three sources of high energy electrons to the electron transport chain. As mentioned previously, the Krebs cycle also produces reduced FAD, another electron carrier. At one step in the Krebs cycle, succinate is transformed into fumarate by Succinate Dehydrogenase. Succinate Dehydrogenase is bound up on the inner membrane, the only enzyme in the Krebs cycle that isn’t free floating in the matrix space. FAD (and when reduced FADH2) is complexed with Succinate Dehydrogenase, and together, they form the aforementioned Complex 2. Electrons entering the transport chain through complex 2 are of lower energy than electrons entering the cycle through NADH. Thus electrons from FADH2 are transferred directly to Ubiquinone, and do not pass through complex 1. From then on the cycle is the same as for electrons from NADH. Thus for each molecule of FAD reduced, six protons are pumped across the inner membrane (four from complex 3, and two from complex 4).
The third source of high energy electrons is from cytosolic NADH. For each molecule of glucose that is processed by glycolysis there are two molecules of NADH that are produced. Most of this cytosolic NADH gets used up in cytosolic processes, however some of it is transported into the mitochondria for use in the electron transport chain. However, since it has to be transported across the inner membrane (it can simply float through the porins in the outer membrane), its electrons loose some of their energy. Specifically, electrons from cytosolic NADH are transported into the electron transport chain via the glycerol phosphate shuttle. This process uses the electrons on the cytosolic NADH to reduce FAD, producing FADH2. These electrons then enter the transport chain via ubiquinone, and have the same contribution per cytosolic NADH as per mitochondrial FADH2.
The net effect of this electron transport chain is to create a gradient in charge, pH and concentration of hydrogen ions. There is a tenfold concentration of protons on the outside of the matrix space, leading to the matrix space having a pH of approximately 8 (the intermembrane space is for all intents and purposes continuous with the cytosol, so its pH does not drop). Furthermore, from the concentration of hydroxide on the inside and the resulting high concentration of ATP, there is charge gradient across the inner membrane of 200 mV. These three gradients together form the proton motive force, which is then used to drive ADP phosphorylation.
The proton motive force is also used to generate heat. In brown fat, (a high density fat possessed by infants and animals living in very cold environments. Also referred to as baby fat.) the proton gradient is expended by special hydrogen ion channels that let the protons back in. This releases energy, creating heat. This heat is used to protect organisms particularly sensitive to heat changes.
![]() |
Add caption |
Protons are guided back into the matrix space primarily via ATP Synthase (also called ATPase). ATPase has two major subunits, F0 which is lodged in the inner mitochondrial membrane, and F1 which protrudes into the matrix space. F0 unit grabs onto a proton and ligates it to one of the 10-14 identical proteins that make up a rotor. This then causes the rotor to spin. A proton is carried around for one full spin, after which it is deposited upon the inside of the membrane. The spinning rotor turns inside the F1 subunit, which does not spin. Thus ATPase changes the chemical energy of the the proton motive force into the mechanical energy of the spinning rotor. It is this mechanical energy that brings ADP and inorganic phosphate into correct alignment to produce ATP. It requires between 2 and 3 Hydrogen ions to catalyze the production of 1 molecule of ATP. This means that each NADH has enough energy to make about 2.5 molecules of ATP from ADP through oxidative phosphorylation. All told, this yields around 30 molecules of ATP from 1 molecule of glucose, when the 2 ATP’s from Glycolysis are added in.
If you found this description confusing, check out this movie in which an actin fiber has been connected to the spinning rotor. You can also check out this movie or this one:
So, to sum it all up:
Well that is all for this post. Thanks for stopping by. The information for this post was attained from Alberts’ Molecular Biology of the Cell, and the Friday, October 19th lecture by Dr. Todd Rosentiel.
Previous Relevant Posts: Mitochondria Pt. 1 — Structure